Protein C (autoprothrombin IIA, blood coagulation factor XIV) is a zymogen, the activated form of which plays an important role in regulating anticoagulation, inflammation, cell death, and maintaining the permeability of blood vessel walls in humans and other animals. Activated protein C (APC) is a serine protease that proteolytically inactivates proteins Factor Va and Factor VIIIa.
The zymogenic form of protein C is a vitamin K-dependent glycoprotein that circulates in blood plasma. Its structure is that of a two-chain polypeptide consisting of a light chain and a heavy chain connected by a disulfide bond. Protein C is activated when it binds to thrombin and is greatly promoted by the presence of thrombomodulin and endothelial protein C receptors (EPCRs). Because of EPCR’s role, activated protein C is found primarily near endothelial cells (those that make up the walls of blood vessels), and it is these cells and leukocytes (white blood cells) that APC affects. Because of the crucial role that protein C plays as an anticoagulant, those with deficiencies in protein C, or some kind of resistance to APC, suffer from a significantly increased risk of forming dangerous blood clots (thrombosis).
Human protein C is structurally similar to other vitamin K-dependent proteins affecting blood clotting, such as prothrombin, Factor VII, Factor IX and Factor X.
Protein C is a major component in anticoagulation in the human body. APC proteolyses peptide bonds in activated Factor V and Factor VIII (Factor Va and Factor VIIIa), and one of the amino acids in the bond is serine. These proteins that APC inactivates, Factor Va and Factor VIIIa, are highly procoagulant cofactors in the generation of thrombin, which is a crucial element in blood clotting; together they are part of the prothrombinase complex. Cofactors in the inactivation of Factor Va and Factor VIIIa include protein S, Factor V, high-density lipoprotein, anionic phospholipids and glycosphingolipids.
The blood coagulation system is carefully controlled in vivo by several anticoagulant mechanisms, which ensure that clot propagation does not lead to occlusion of the vasculature. The protein C pathway is one of these anticoagulant systems. During the last few years it has been found that inherited defects of the protein C system are underlying risk factors in a majority of cases with familial thrombophilia. The factor V gene mutation recently identified in conjunction with APC resistance is such a defect which, in combination with protein C deficiency, increases the thrombosis risk considerably.
Determination of Protein C Activity with Snake Venom and S-2366™
Introduction
Protein C is vitamin K-dependent inactive precursor of a serine protease (APC), which is the key component in a physiologically important natural anticoagulant system. Genetic defects associated with this system are the major underlying cause of familial thrombophilia. This monograph reviews the biochemistry and clinical implications of protein C and presents a functional protein C assay using a snake venom activator and the chromogenic substrate S-2366™. Blood coagulation is an enzymatic event initiated in response to tissue damage. The binding of coagulation factor VII to exposed tissue-factor starts a cascade of reactions that ultimately leads to the formation of thrombin. Thrombin is the central enzyme in the coagulation system. It clots blood by converting soluble fibrinogen to clot-forming fibrin monomers and activates factor XIII leading to the strengthening of the clot by cross-linking. Thrombin also provides a link between the coagulation cascade and cellmediated hemostasis by activating platelets. Furthermore, via a positive feed-back reaction thrombin accelerates its own generation by the activation of factors V and VIII. These proteins bind to negatively charged phospholipids on the surface of activated platelets and serve as cofactors for factors IXa and Xa, respectively. The total effect is a dramatic burst of thrombin and resulting fibrin deposition at the site of injury.
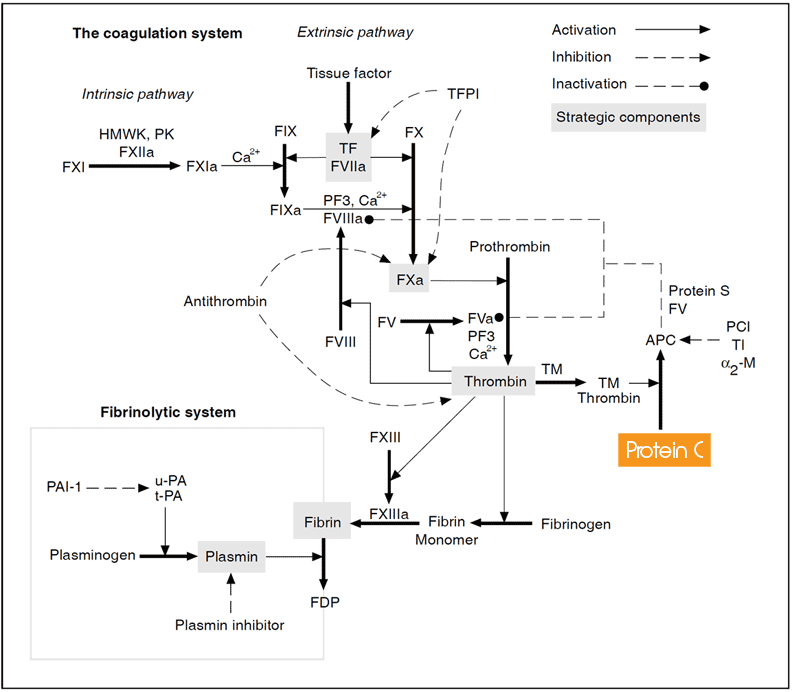
The coagulation system. Coagulation factors are represented by their Roman numerals (a = activated). Abbreviations: HMWK = high molecular weight kininogen, PK = prekallikrein, K = kallikrein, TF = tissue factor, TFPI = tissue factor pathway inhibitor, PF3 = phospholipid, TM = thrombomodulin, PC = protein C, APC = activated protein C, PCI = protein C inhibitor, TI = trypsin inhibitor, a2M = a2-macroglobulin, FDP = fibrin degradation products.
Coagulation factors and regulatory proteins
Factor | Name | Size [kDa] | Concentration [mg/ml] |
I | Fibrinogen | 340 | 3000 |
II | Prothrombin | 69 | 100 |
III | Tissue factor | 47 | – |
IV | Calcium | – | – |
V | Proaccelerin | 330 | 10 |
VI | – | – | – |
VII | Proconvertin | 48 | 0.5 |
VIII | Antihemophilic factor | 330 | 0.1 |
IX | Christmas factor | 55 | 5 |
X | Stuart-Prower factor | 59 | 8 |
XI | Thromboplastin antecedent | 160 | 5 |
XII | Hageman factor | 80 | 30 |
XIII | Fibrin-stabilizing | 320 | 10 |
– | Tissue factor | 37 | – |
– | Antithrombin | 58 | 150 |
– | Heparin cofactor II | 66 | 91 |
It is obvious that the autocatalytic nature of thrombin would, if unchecked, clot the blood content of a person within minutes. Anticoagulant control in humans is primarily achieved by two principally different mechanisms. A direct type of regulation is provided by a group of circulating enzyme inhibitors of which the two, antithrombin and the tissue factor pathway inhibitor, are of particular importance. The second type of anticoagulant mechanism is associated with intact blood vessels and is initiated by thrombin itself. When thrombin binds to the protein thrombomodulin on the surface of endothelial cells, a remarkable transformation takes place that removes most of its procoagulant functions. Instead, thrombin becomes a potent activator of protein C, the key component in the protein C anticoagulant system. Together with protein S and factor V as potentiating cofactors, the activated protein C (APC) effectively degrades membrane-bound factors Va and VIIIa, thus limiting further coagulation.
The physiological importance of the protein C anticoagulant system is demonstrated clinically by the diseases associated with it. Severe thrombosis known as purpura fulminans may occur in neonates with a rare homozygous protein C deficiency, whereas individuals with the more common heterozygous protein C deficiency may suffer an increased risk of venous thrombosis later in life. Inherited protein S deficiency has a similar risk and is found to be equally frequent, about 2-5%, in cohorts of thrombosis patients.Of great interest recently is the described mutation in the factor V gene. The defect results in resistance to APC and is reported to be the major cause of familial thrombosis.
Schematic illustration of the protein C anticoagulant pathway
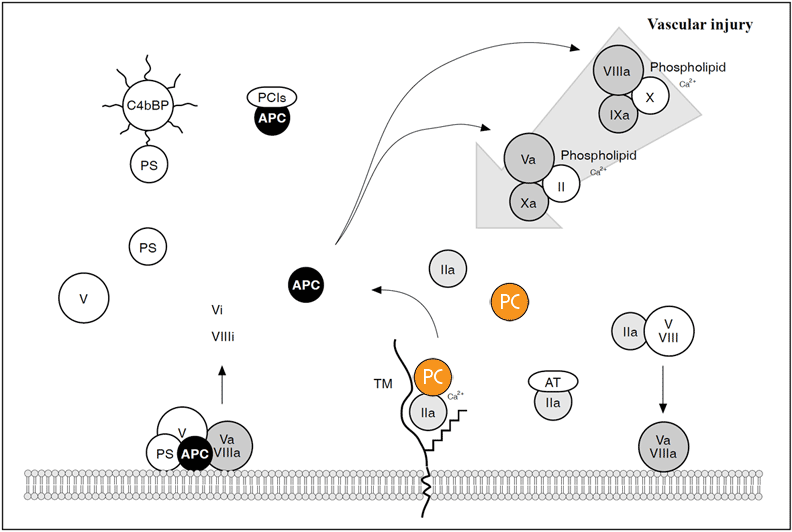
Thrombin (IIa) escaping the site of vascular injury binds to a high affinity receptor called thrombomodulin (TM), located on the surface of intact endothelial cells. Thrombin bound to TM looses most of its procoagulant properties instead, it becomes a potent activator of protein C in the presence of calcium ions. Activated protein C (APC) specifically degrades the membrane-bound factors Va and VIIIa, whereas non-activated factors V and VIII are poor substrates for APC. The activity of APC is potentiated by protein S and factor V. These two plasma proteins function in synergy as membrane-bound cofactors to APC and probably also, localize and focus the APC activity to the surface of endothelium and platelets. About 60% of protein S in plasma circulates in a complex with the octupus-shaped C4b-binding protein (C4bBP). Only free protein S functions as a cofactor for APC. APC is slowly neutralized by protein C inhibitors (PCIs). Thrombin bound to TM will be inhibited either by antithrombin (AT) or by endocytosis of the thrombin/TM complex.
Major components involved in the human protein C anticoagulant system
Component | Size [kDa] | Concentration [mg/ml] | Function |
Protein C | 62 | 3-5 | Zymogen activated by thrombin/TM |
Activated protein C | – | – | Cleaves/inactivates factors Va, VIIIa |
Protein S | 71 | 20-25 | Cofactor for APC |
Factor V | 350 | 10 | Cofactor for APC |
Thrombomodulin | 60 | 0,02* | Cofactor for protein C activation |
Protein C inhibitor | 57 | 5 | Specific inhibitor of APC |
C4b-binding protein | 570 | 150 | Binds to protein S |
* Soluble thrombomodulin |
The laboratory evaluation of this natural anticoagulant system is clearly of utmost importance. Herein we describe various assay methods for protein C and present the assay kit Chromogenix Coamatic® protein C, highly suitable for the routine clinical laboratory.
Protein C data
Name: | Protein C |
Synonyms: | Autoprothrombin II, PC |
History: | Described by Seegers et al in 1960, isolated/identified by Stenflo in 1976 |
Concentration: | 3-5 µg/ml |
Molecular weight: | 62,000 daltons |
Primary structure: | 461 amino acids |
Carbohydrate: | 23% |
Half-life: | 6-8 hours |
Gene: | 11 kb, chromosome 2, position q14-q21, composed of 9 exons and 8 introns |
Type: | Vitamin K-dependent zymogen of a serine protease |
Function: | APC degrades factors Va and VIIIa |
Importance: | Hereditary deficiency of PC is a risk factor for venous thromboembolism |
Protein C biochemistry
Structure and functional aspects
Protein C is synthesized in the liver as a 461 amino acids long single chain precursor. Prior to secretion, it is processed into a disulfide-linked two chain polypeptide containing, one light (155 amino acids) and one heavy chain (262 amino acids). The molecular weight of the mature protein is approximately 62,000 daltons and its carbohydrate content about 23%. It circulates in human plasma at a concentration of 3-5 mg/ml and its half-life is 6-8 hours. Protein C is an inactive pro-enzyme or zymogen of a serine protease (APC).
From information based upon the protein C gene structure and amino acid sequence homologies with other proteins, it is clear that protein C contains several regions with discrete structural or functional properties.
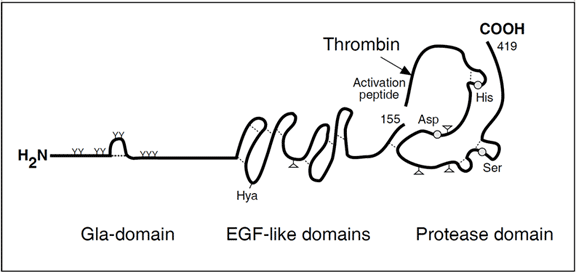
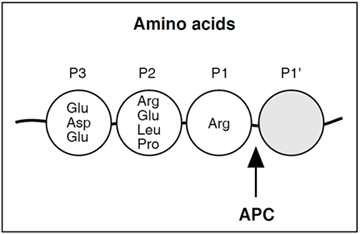
The amino terminal light chain consists of a Gla domain, with nine glutamic acid residues that are g- carboxylated in a vitamin K-dependent reaction in the liver. This region interacts with negatively charged phospholipid in the presence of calcium ions (Ca2+) and is a prerequisite for the anticoagulant function of APC. The Gla-domain is followed by two EGF-like domains, which appear to interact with protein S. Together with the Gla-domain these domains are also important for the binding of protein C to the thrombin-thrombomodulin complex.
The heavy chain contains the serine protease domain with the ‘catalytic triad’ composed of histidine, aspartic acid and serine (located at position 211, 257 and 360, respectively). These amino acid residues are situated relatively far apart from each other in the primary structure, but are in close proximity in the folded protein. APC cleaves substrates with arginine in the P1 position, whereas the amino acid composition in the P2 and P3 positions are more varied.
It is not known which structural features give APC its narrow specificity for factors Va and VIIIa although, it probably involves a secondary binding site located outside the catalytic center. Recent studies using synthetic peptides indicate that the amino acid residues 311- 325 and 390-404 in APC are important for its anticoagulant interaction with substrates.
Protein C activation
Protein C must be transformed to an active serine protease to be physiologically functional. The activation of human protein C occurs through the enzymatic removal of a small activation peptide from the aminoterminus of the heavy chain. In vivo, the activation is mediated by the thrombin-thrombomodulin complex. In vitro, the activation can be achieved using thrombin alone, trypsin, or proteases from various snake venoms.
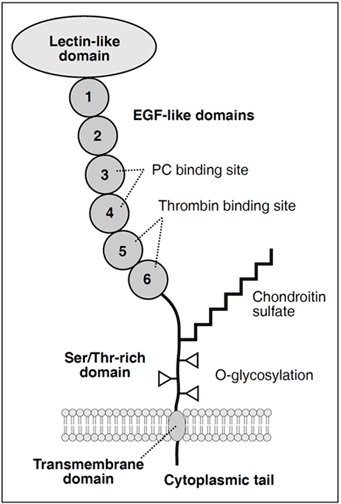
Thrombin-Thrombomodulin
Activation of protein C by thrombin alone is slow and has no physiological function. However, when thrombin binds to the integral membrane protein thrombomodulin (TM), present on the vascular endothelium, the result is a 20,000-fold increase in the rate by which thrombin activates protein C. TM also removes the procoagulant properties of thrombin and accelerates the thrombin-antithrombin reaction by a glycosaminoglycan moiety, identified as chondroitin sulfate. Ca2+ is an important regulator that enhances protein C activation by the thrombin-TM complex, but inhibits the activation by thrombin.
The mature TM molecule consists of 557 amino acids residues arranged in a lectin-like domain, six EGF-like domains, a Ser/Thr-rich domain, a transmembrane domain, and a short cytoplasmic tail.
TM has a widespread distribution in the mammalian organism and with few exceptions, is expressed by endothelial cells of arteries, veins, capillaries, and lymphatic vessels. The highest concentration of TM is found in the capillaries, where the ratio of endothelial surface to the volume of circulating blood is high. Thrombin entering the microcirculation will therefore be extracted rapidly by TM and protein C will be activated. This is physiologically important as even small amounts of thrombin would generate enough fibrin to stop the blood flow in the microvasculature.
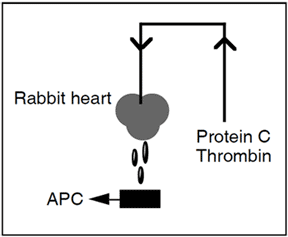
In the experiments that lead to the discovery of thrombomodulin (Esmon and Owen 1981), the capillary bed of a rabbit heart (a so-called Langendorff heart preparation) was perfused with protein C and thrombin. Protein C was rapidly activated by thrombin bound to a high affinity receptor on the endothelium. This receptor was named thrombomodulin since it dramatically modulated the substrate specificity of thrombin.
Snake venoms
A number of snake venoms have been reported to contain protein C activating activity particulary from the Agkistrodon genus. Three serine proteases have been isolated and characterized from venoms of this family including the species of A. bilineatus, A. halys halys, and A. contortrix contortrix. The enzymes are fast-acting specific protein C activators, with a single-chain structure in the molecular weight range of 35,000-38,000 daltons.
Function of APC
The anticoagulant function of APC is demonstrated by prolongation of both the prothrombin time and the activated partial thromboplastin time (APTT). APC cleaves and inactivates two membrane-bound procoagulant proteins, factor Va and factor VIIIa, resulting in decreased thrombin generation. Circulating non-activated forms of these proteins (factors V and VIII) are poor substrates for APC. In addition to its anticoagulant function, it has been suggested that APC possesses profibrinolytic activity.
APC cofactors
The molecular mechanisms involved in the protein C anticoagulant system is highly complex and so far poorly understood. However, essential for the maximal catalytic efficiency of APC is probably the presence of helper proteins or cofactors, which promote the binding of APC to phospholipid on cellular surfaces and give support to the APC degradation of the membrane-bound factors Va and VIIIa. Two cofactors are now associated with APC, one is the recently discovered cofactor-function of factor V, the other is the established cofactor, protein S.
Protein S
Protein S is a vitamin K-dependent plasma protein, which apart from being an APC cofactor binds a regulatory acute-phase protein of the complement system, C4b-binding protein (C4bBP), and mediates its attachment to phospholipid. Approximately 60% of protein S in plasma is bound to C4bBP. Only the free form of protein S has a APC cofactor function. Protein S enhances binding of APC to the phospholipid of platelets, platelet microparticles and endothelial cells. In vitro experiments have shown that protein S abrogates factor Xa-mediated protection of factor Va and factor IXa-mediated protection of factor VIII, making the substrates available for APC.
Factor V
The observation by Dahlbäck et al. suggests a possible anticoagulant role for factor V. It was found that in the presence of both factor V and protein S, APC degrades its substrates efficiently, whereas APC alone or together with factor V was inefficient. The combination with APC and protein S was less potent than when factor V was also present. This suggets that protein S and factor V function as synergistic cofactors to APC in a multimolecular complex on the membrane surface.
APC inhibitors
APC is inhibited relatively slowly by at least three protease inhibitors in plasma, including protein C inhibitor (also known as PAI-3), trypsin inhibitor,24 and a2-macroglobulin. Heparin stimulates only the activity of the protein C inhibitor. The half-life of APC in the circulation is about 10-20 minutes.
Clinical aspects of Protein C
Protein C deficiency
Venous thrombosis is a serious medical problem, which anually affects 1 in 1000 people. The pathogenesis of the disease is very likely multifactoral, involving both circumstantial and genetic risk factors. The importance of identifying the underlying genetic cause has been obvious, as up to 40% of patients with thrombosis have positive family histories. The major hereditary disorders of the coagulation system known to predispose for venous thrombosis, include APC resistance and deficiencies of protein C, protein S, and antithrombin. Together these defects may account for up to 60% of cases with familial thrombophilia or about 30% of unselected thrombosis patients.
Hereditary protein C deficiency
The relevance of evaluating protein C levels in a patient’s plasma became clear in 1981, when the first case of hereditary protein C deficiency associated with thrombotic disease was reported by Griffin et al. Several studies have confirmed this initial report and it has been shown that individuals with an isolated protein C deficiency may run a 6 to 9-fold increased risk for venous thrombosis.
Prevalence of inherited blood protein defects in thrombosis patients
Defect | Prevalence |
---|---|
APC Resistance | 20-30% |
Protein C deficiency | 2-5% |
Protein S deficiency | 2-5% |
Antithrombin deficiency | 1-5% |
Features of hereditary protein C deficiency
Clinical manifestations
- Autosomal dominant
- Venous thrombosis of the lower limbs
- Occurence of thrombosis in the microcirculation
- 50% of first events begin before the age of 30-45
- Oral anticoagulant-induced skin necrosis
Laboratory diagnosis
- Low protein C activity levels (< 70% of normal)
- Low antigen levels (type II)
- Normal antigen levels (type I)
Treatment options
- Warfarin or heparin for long-term treatment
- Heparin for acute thrombotic events
- Protein C concentrates for homozygotes
Protein C levels
Hereditary protein C deficiency is inherited as an autosomal dominant trait. Heterozygotes for protein C deficiency have protein C activity or antigen levels of 30 to 70% of normal, whereas homozygotes (or compound heterozygotes) with a severe defect have levels below 1%. Homozygotes with a mild defect have also been reported with protein C levels of 10-24%. The normal range of protein C in the adult is 70% to 130% of a normal plasma pool (defined as 100%).
Prevalence
The prevalence of protein C deficiency is 2-5% in patients with thromboembolic disease. Selected patient-groups with thrombosis occurring at a young age tend to have a higher prevalence (up to 10-15%). Extrapolation into the general population would give an estimate prevalence for symptomatic protein C deficiency of one in 20,000 people. However, the identification of symptom-free individuals with protein C deficiency in large groups of healthy blood donors put the prevalence at between one in 200 and one in 500. The majority of these individuals did not present family histories of thrombosis. It remains to be established whether clinical phenotypes reflect different defects in the protein C gene or if additional risk factors, genetic or circumstantial, are required for expression of the symptomatic phenotype.
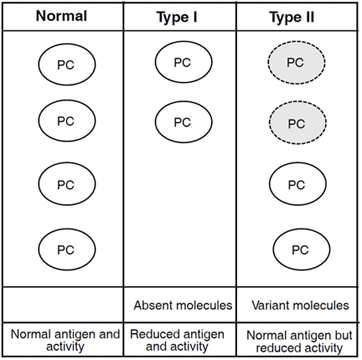
Classification
Two types of protein C deficiency states are recognized. In type I deficiency the plasma concentration of protein C is reduced both in functional and immunological assays, reflecting a genetic defect causing a reduced biosynthesis of protein C. Type II deficiency is characterized by normal protein C antigen levels, but with decreased functional activity. This type of defect reflects the synthesis of abnormal molecules with reduced function. Type I deficiency is the most common type of disorder. The mutations in the protein C gene have been characterized in a recently published database.
Clinical features
The most common clinical manifestation of symptomatic heterozygous protein C deficiency is deep venous thrombosis of the lower extremities. The first thrombotic event usually occurs after puberty. Of the affected patients, 50-80% experience the event before the age of 30-45. Important circumstantial risk factors known to be associated with the apperance of thrombosis include surgery, immobilization, pregnancy and oral contraceptives. However, approximately 50% of all the first thrombotic events and 65% of recurrences occur spontaneously without apparent cause.
In contrast to the heterozygous state, which by itself is a relatively weak risk factor for thrombosis, patients with homozygous protein C deficiency usually suffer from severe and fatal thrombosis in the early stage of life. The clinical picture, known as purpura fulminans, is associated with skin necrosis (due to microvascular thrombosis), thrombosis in the brain, and DIC. The incidence of homozygous or compound heterozygous protein C deficiency is extremly rare (1 in 500,000 births).
Common risk factors associated with deep venous thrombosis (DVT)
- Surgery
- Increasing age
- Malignancy
- Immobilization
- Heart failure or myocardial infarction
- Previous DVT
- Obesity
- Pregnancy
- Oral contraceptives
- Stroke with limb paresis or paralysis
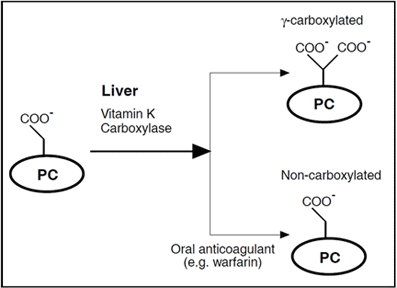
Drug-induced skin necrosis
Protein C deficiency is associated with an increased risk of developing skin necrosis in the initial phase of oral anticoagulant therapy with vitamin K-antagonists such as warfarin sodium and bishydroxycoumarin. The drugs interfere in the vitamin K-dependent biosynthesis of several proteins in the coagulation system (e.g protein C, protein S, prothrombin, factor VII, factor IX, factor X). Skin necrosis is thought to develop as a result of the relatively short biological half-life (t1/2 = 6–8 hours) of protein C compared to those of other vitamin K dependent coagulation factors (t1/2 ›40 hours). During the initial phase of oral anticoagulant therapy this may lead to a sharp decline in protein C activity levels, causing a transient but severe hypercoagulable state.
Acquired protein C deficiency
The Protein C level is influenced by various diseases and drugs. Acquired protein C deficiency is often associated with disseminated intravascular coagulation (DIC), deep vein thrombosis, severe liver disease, sepsis, vitamin K deficiency, oral anticoagulant therapy and elective surgery. The protein C activity level may in some cases indicate the severity of a disease and can be used as a prognostic parameter.
Conditions and drugs associated with an elevated or decreased protein C level
Decreased levels
- DIC
- Deep vein thrombosis
- Pulmonary embolus
- Severe liver disease
- Post-operative patients
- Infection
- Malignancy
- L-asparaginase therapy
- Adult respiratory distress syndrome
- Hemolytic uremic syndrome
- Thrombotic thrombocytopenic purpura
- Oral anticoagulants
- Vitamin K-deficiency
- Neonatal period
Elevated PC levels
- Diabetes
- Nephrotic syndrome
- Late pregnancy
- Oral contraceptives
- Anabolic steroids
Elevated protein C levels
Elevated protein C levels have been reported in diabetic and nephrotic patients, during late pregnancy, and with oral contraceptives and anabolic steroids. Elevated levels have no known clinical significance. However, clinical conditions and drugs influencing the protein C level should always be considered for the correct laboratory diagnosis of hereditary protein C deficiency.
Treatment options
Treatments for protein C deficiency include heparin, warfarin, and protein C concentrates. Acute thrombosis in protein C-deficient individuals should be treated with heparin. Warfarin is used for longer treatment periods to prevent thrombotic recurrences. When initiating the warfarin therapy it must be started at low doses in conjunction with heparin to prevent skin and fat necrosis.
Prophylactic anticoagulation is mainly recommended for symptomatic patients in high risk situations (e.g. surgery, pregnancy). Symptom-free relatives of symptomatic patients with protein C deficiency may benefit from prophylactic anticoagulation in similar risk situations, since they run an increased risk of thrombosis compared to nondeficient individuals.
Replacement of protein C can be carried out with fresh frozen plasma or pure protein C concentrates produced either from plasma or by recombinant techniques. There is currently major interest in the use of APC preparations in the management of acute DIC. Studies of APC in animal models show that it is a powerful antithrombotic agent, without the risk of bleeding as a side effect.
Protein C Assay methods
Protein C assays
The laboratory evaluation of protein C is the only definitive way of diagnosing hereditary protein C deficiency in thrombophilic patients. Various types of assays have been developed and some are available in commercial kit form.
Protein C is measured using either a functional assay, that tries to evaluate the biological activity of protein C, or an immunological assay, which determines the total amount of protein C related material in plasma. Each assay has a number of pros and cons. However, for the routine screening of hereditary protein C deficiency, a functional activity assay is generally recommended. This approach will detect low activity levels associated with both reduced (type I) as well as dysfunctional protein C (type II).
Activity assays
Numerous activity assays have been described that use different types of activators and detection methodologies. The majority of the proposed methods can be divided into three major steps: (1) isolation of protein C from plasma, (2) protein C activation, and (3) measurement of APC using either synthetic substrates or clotting-based assays.
Assay | Step 1Separation of PC from interfering substances | Step 2PC Activator | Step 3APC activity measurement |
Bertina et al. | Al(OH) | Thrombin | S-2366™ |
Sala et al. | Barium citrate | Thrombin/TM | S-2266™ |
Francis & Patch. | Barium citrate | Thrombin | APTT |
Francis. | Barium citrate | Thrombin/TM | APTT |
Vigano et al. | Monoclonal antibody | Thrombin/TM | S-2238™ or FXa clot. |
Martinolo & Stocker. | – | Protac® | APTT |
Gugliemone & Vides. | – | Protac® | PT |
McCall et al. | – | Protac® | S-2366™ |
Odegaard et al. | – | Protac® | S-2366™ |
Functional assays for protein C involve three major procedures: isolation of protein C, activation of protein C and measurement of protein C activity. At least one step from each group is necessary to develop a functional assay. The use of Protac® negates the need for an isolation step. APTT = activated partial thromboplastin time, PT = prothrombin time.
Isolation step
In the first generation of functional protein C assays the activation of protein C was achieved either by thrombin alone, or by the thrombin-thrombomodulin complex. These reagents required an adsorption step prior to the protein C activation in order to isolate protein C from its plasma inhibitors and other interfering substances. The surface binding of protein C was obtained using either immunoadsorption techniques or insoluble salts (e.g barium citrate or aluminium hydroxide). The latter procedure exploited the ability of protein C and other vitamin K-dependent proteins to bind to insoluble salts via the Gla-domain. Once protein C was isolated and activated, the thrombin excess has to be quenched or removed by specific thrombin inhibitors (e.g. I-2581, antithrombin) before the protein C activity could be quantified accurately. In general, these multi-step assays have been shown to be specific for protein C but are time-consuming and unsuitable for clinical use.
Snake venom activator
The determination of protein C was greatly facilitatedby the use of a specific protein C activator with the trade name Protac®. The activator is a serine protease isolated and purified from the venom of the southern copperhead snake, Agkistrodon c. contortrix. It rapidly activates both human and bovine protein C, probably via the same mechanism as thrombin, without interfering with other coagulation factors. The activation reaction is especially effective in the absence of calcium ions and conditions of low ionic strength. Furthermore, the venom activator does not hydrolyze the chromogenic substrates for protein C to any significant extent. Since the activation is rapid, it minimizes the efficiency of the protein C inhibitors and thus eliminates the need for isolating protein C in an adsorption step.
Measurement of APC
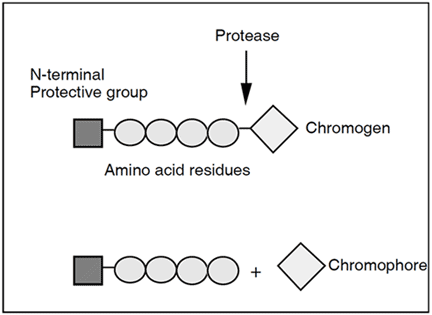
APC can be measured using either chromogenic substrates or clotting-based techniques.
Chromogenic substrates are small synthetic peptides that mimic the cleavage site of a natural substrate. The peptides are generally composed of a sequence of 2-4 amino acids with the chromogen, 4-nitroaniline (pNA) attached to the end. When the chromogenic substrate is incubated with a proteolytic enzyme, such as APC, it is cleaved and pNA (yellow color) is released. The release is measured at a wavelength of 405 nm, either during the reaction in a photometer cuvette (kinetic method), or discontinuously by stopping the reaction with acetic or citric acid (end-point method). The photometric signal is proportional to the enzyme activity in a properly-designed assay.
Substrates to be used in a chromogenic assay for protein C must be specific for the enzyme, and activators and contaminating factors should not cleave the substrate. One of the most suitable chromogenic substrate available for the assay of protein C activated by Protac®, appears to be Chromogenix S-2366™. The substrate has been shown to have little sensitivity to the isolated venom activator, although it is significantly hydrolyzed by normal plasma mixed with the activator.
Clotting assays for the determination of protein C use the ability of activated protein C to prolong the clotting time. A widely-used method is the activated partial thromboplastin time, APTT. The use of a protein C-deficient plasma in these assays negates the possibility that deficiencies of other plasma proteins (e.g. protein S) are the cause of APTT prolongation. APTT assays for protein C are generally less precise and show a greater variability than chromogenic protein C assays. This is probably due to the influence of high levels of factor VIII (an acute phase protein), and/or the variable phospholipid reagent quality and composition. The interference may result in erroneously low protein C levels. Since the APTT assays are based on APC’s ability to inactivate factors Va and VIIa in the natural environment, they offer the possibility of evaluating all the functions of the protein C molecule. This is important for a small minority of patients that have defects in the protein molecule not associated with amidolytic functions.
Chromogenic substrates
- Chromogenix S-2366™ pyroGlu-Pro-Arg-pNA
- Chromogenix S-2266™ H-D-Val-Leu-Arg-pNA
- Chromogenix S-2288™ H-D-Ile-Pro-Arg-pNA
- Chromogenix S-2238™ H-D-Phe-Pip-Arg-pNA
Chromogenic substrates used in amidolytic protein C assays.
Criteria for optimal function of protein C
- Expression of normal antigen concentration (70-130%)
- Presence of Gla residues, calcium-binding
- Activation by the thrombin-thrombomodulin complex
- Interaction with phospholipids and protein S/factor V
- Recognition and degradation of factors Va and VIIIa
- Inhibition by protein C inhibitors
Immunological assays
Immunological assays for protein C are usually based on the use of monoclonal antibodies against protein C and include electroimmunoassays (EIA), radioimmunoassays (RIA), and enzyme-linked immunosorbent assays, ELISA. The advantages of these assays are their specificity, reproducibility and accuracy. However, since immunological assays measure all types of protein C molecules in plasma without evaluating their function, they will not detect dysfunctional molecules (type II deficiency).
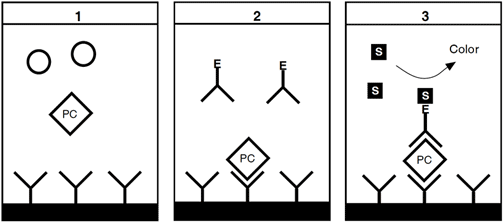
Enzyme-linked immunosorbent assayProtein C assay of the ELISA type are often commercially available. In general, a support is coated with protein C-specific antibody fragments. [1] The protein C antigen in the plasma sample is allowed to react with the antibody, followed by a washing step. [2] A conjugate (enzyme-labeled anti-protein C antibodies) is then added, forming a sandwich-like complex in the presence of protein C antigen. This is followed by a second washing. [3] Enzyme activity, proportional to protein C concentration, is determined by the cleavage of a substrate yielding a colored or fluorescent product.
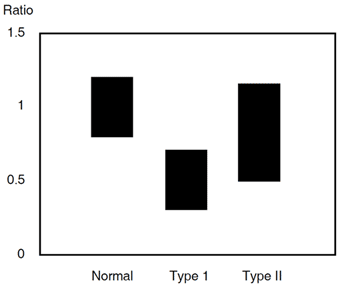
Laboratory aspects
Oral anticoagulant therapy
The diagnosis of hereditary protein C deficiency is particularly problematic in patients on oral anticoagulant (OAC) therapy. As explained previously, OAC therapy interferes with the formation of the g-carboxyglutamic acid moiety of the protein C molecules during biosynthesis in the liver, which results in a loss of anticoagulant activity. Noncarboxylated forms of protein C molecules that are inactive in vivo can however, still be activated by snake venoms or thrombin-thrombomodulin and retain amidolytic activity in vitro. Assays using chromogenic substrates will therefore over-estimate the true level of protein C activity in plasma from patients receiving OACs.
Theoretically, clotting-based assays would be preferable to use in patients on OAC treatment since they evaluate all of the functional domains of the APC molecule. However, clotting techniques have the principal disadvantage that protein C activity levels in all patients are very low, which makes it difficult to identify individuals with an inherited deficency. Certain statistical procedures may minimize this problem. Ratios of protein C activity to thrombin activity have been used to improve the discrimination between deficient and non-deficient patients on OAC therapy.
Antigen assays are also difficult to interpretate in patients on OAC therapy, in that the loss of anticoagulant activity is more marked than the corresponding decrease in antigenic level. This antigen to activity ratio and its discrepancy during OAC therapy should always be considered when the appropriate immunological assay is to be chosen. Ratios of protein C antigen to factor X antigen and/or protein C antigen to factor II antigen are often used to determine a quantitative type I deficency in patients on OAC therapy.
Contact factor proteases
In plasmas from patients on streptokinase therapy and in plasmas where contact activation is suspected, such as with DIC patients or individuals on oral contraceptives where cold activation may occur, there is a contribution to the chromogenic substrate cleavage that is not associated with the activity of protein C. This is because all known chromogenic protein C substrates are susceptible to some degree to activities generated in the contact activation system. To avoid the subsequent risk of overestimating the protein C levels in such plasmas it is recommended that the substrate blank activity, in the absence of Protac®, is measured and used for correction of the results.
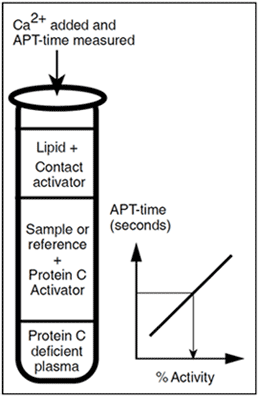
Protein C Products
The development of the first chromogenic peptide substrate S-2160™ in the early Seventies, initiated the introduction of photometry in hematology. Today, Chromogenix has a wide range of chromogenic peptide substrates as well as complete assay kits with applications extending from routine analysis to front-line research in both coagulation and fibrinolysis. The tests can be performed manually or on automated analytical systems with high specificity, sensitivity and accuracy. Important, non-chromogenic based products include APTT-test for APC resistance.
Chromogenic kit
Chromogenix Coamatic® Protein C is a chromogenic substrate assay for the measurement of protein C activity in human plasma. It is easy to perform and is suitable for application in the majority of automated instruments. The kit contains a specific and rapid protein C activator (Protac®) from the venom of Akgistrodon c. contortrix, and the highly-sensitive and specific chromogenic substrate S-2366™.
Measurement principle
Protein C is activated by a thrombin-like enzyme, Protac®, from the venom of the southern copperhead (Agkistrodon contorix contorix) and the amount of activated protein C is determined by the rate of hydrolysis of the chromogenic substrate S-2366™. The pNA release measured at 405 nm is proportional to the protein C level up to 120% of normal plasma.
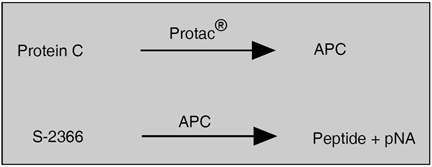
Specificity and interfering factors
There is no interference of heparin levels £ 3 IU/ml. Sample blank activities should be determined and subtracted in plasmas from patients on streptokinase therapy as well as in plasmas where contact factor activation is suspected, e.g. in plasmas from DIC patients or individuals on oral contraceptives susceptible to cold activation. Aprotinin therapy may cause an underestimation of protein C levels.
Assay procedure
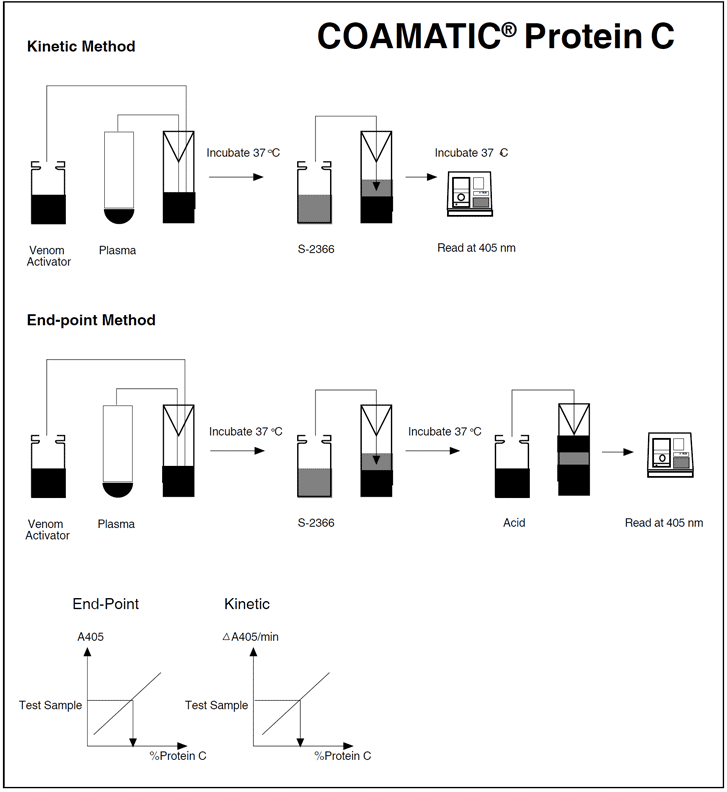
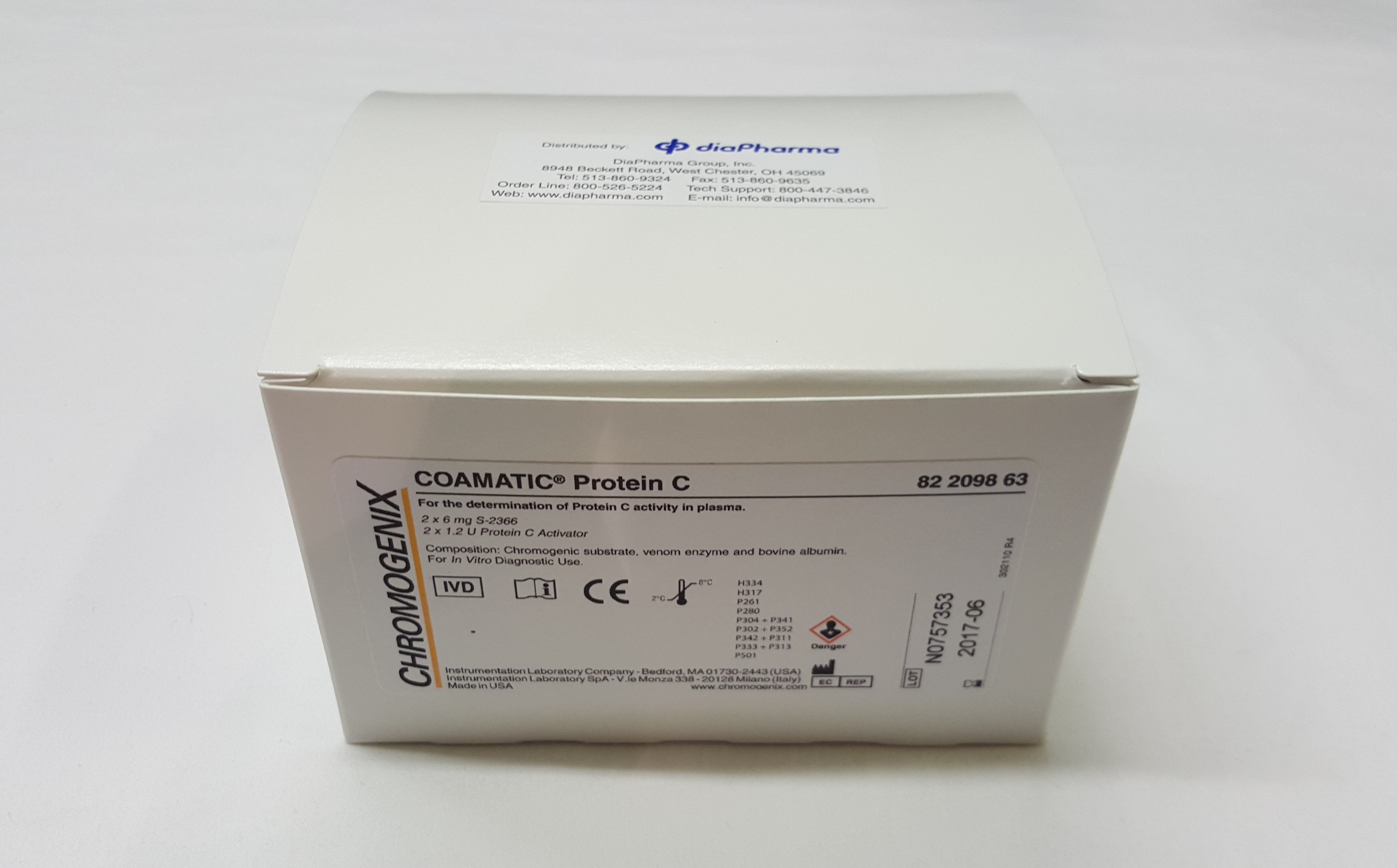