Plasmin is released as a zymogen called plasminogen (PLG) from the liver into the factor IX systemic circulation. Two major glycoforms of plasminogen are present in humans – type I plasminogen contains two glycosylation moieties (N-linked to N289 and O-linked to T346), whereas type II plasminogen contains only a single O-linked sugar (O-linked to T346). Type II plasminogen is preferentially recruited to the cell surface over the type I glycoform. Conversely, type I plasminogen appears more readily recruited to blood clots.
Chromogenic Substrate Assays for Plasminogen and their Relevance
Introduction
Plasminogen is the inactive precursor of plasmin, a potent serine protease involved in the dissolution of fibrin blood clots. Both hereditary and acquired forms of plasminogen deficiency have been described. These are usually associated with either a thrombotic or a hyperfibrinolytic condition. In this monograph we review the plasminogen-plasmin system and describe two chromogenic-based assay kits for the photometric determination of plasminogen activity in human plasma.
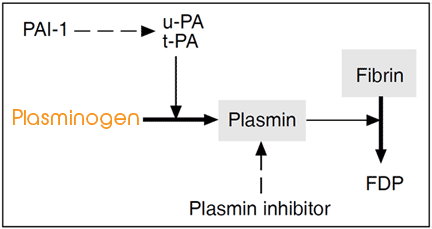
Blood coagulation is a complex enzymatic event culminating in the formation of an insoluble threadlike protein called fibrin. Together with platelets, fibrin forms a hemostatic plug to prevent excessive bleeding. Fibrin blood clots are ultimately dissolved in due course in order to restore vascular patency. The enzymes involved in this physiologically important process are part of the fibrinolytic system.
The central component in the fibrinolytic system is the glycoprotein plasminogen, which is produced by the liver and is present in plasma and most extravascular fluids. Plasminogen is a precursor enzyme (zymogen) which, following partial cleavage by a plasminogen activator is converted to its active and proteolytic form, plasmin. Its primary target is fibrin, but it is also able to degrade several constituents of the extracellular matrix and to convert a number of pro-hormones and cytokine precursors to their active form. Plasmin also appears to be involved in the metastatic spread of cancer.
The generation of plasmin occurs preferentially on the fibrin surface, which offers binding sites for plasminogen and its principle activator in blood, t-PA. This binding stimulates plasminogen activation, but also localizes the action of plasmin to sites of fibrin formation which promotes efficient clot lysis. Further regulation is provided by the presence in plasma of inhibitors, primarily the plasmin inhibitor and the plasminogen activator inhibitor 1 (PAI-1).
The important role of plasminogen in fibrinolyis makes it an interesting parameter to evaluate in various diseases. A decreased plasminogen level may in some situations compromise the body’s ability to degrade fibrin and as such predispose to thrombosis. Hereditary plasminogen deficiency, as a cause of thrombosis, have also been reported in several cases. However, plasminogen deficiency is usually an acquired condition and since plasminogen is the inactive precursor of plasmin, most acquired defects are found in situations with increased fibrinolytic activity. An acquired deficiency is often seen with severe liver disease and acute disseminated intravascular coagulation (DIC), or as a result of thrombolytic therapy with plasminogen activators.
Plasminogen facts
Name: | Plasminogen |
---|---|
Synonym: | Profibrionolysin |
History: | The existence of plg. postulated by Dastre in 1938, identified by Christensen in 1945 purified by Kline 1953 |
Biosynthesis: | Liver |
Concentration: | 200 μg/ml |
Half-life: | 2.2 days (Glu-plg.) 0.8 days (Lys-plg.) |
Molecular weight: | 92.000 daltons |
Carbohydrate: | 2% |
Primary structure: | 791 amino acids |
Function: | Inactive precursor of plasmin |
Type: | Serine protease |
Gene: | Located on chromosome 6, position q26-q27, length 53.5 kb containing 19 exons |
Importance: | Hereditary defects of plasminogen is a predisposing risk factor for thromboembolic disease |
Biochemistry of Plasminogen
The major components of the fibrinolytic system
Compound | Size [kDa] | Amino acids | Plasma concentration | Half-life in circulation | Location of synthesis | Function |
---|---|---|---|---|---|---|
Glu-plasminogen | 92 | 791 | 200 µg/ml | 2.2 days | Liver | Proenzyme of plasmin |
sc.u-PA | 54 | 411 | 8 ng/ml | 5-10 min | Kidney, lung | Plg. activator |
sc.t-PA | 68 | 527 | 5 ng/ml | 3-4 min | Endothelium | Plg. activator |
Plasmin inhibitor | 70 | 452 | 70 µg/ml | 2.6 days | Liver | Plasmin inhibitor |
PAI-1 | 52 | 379 | 20 ng/ml | 2-3 hours | Endothelium | Plg. activator inhibitor |
PAI-2 | 60 | 393 | *250 ng/ml | 24 hours | Placenta | Plg. activator inhibitor |
* 30th week of pregnancy
Evolution of fibrinolytic enzymes
Plasminogen and its natural activators (t-PA, u-PA) belong to a large family of enzymes considered to have evolved from an ancestral protease similar to trypsin- a serine protease of broad specificity that breaks down dietary proteins. The kinship between the fibrinolytic enzymes in question and trypsin is attributed to their similar protease moiety, which cleaves proteins on the C-terminal side of arginyl and lysyl residues. Stretches of high homology are generally found in the active site pocket composed of serine, histidine and aspartic acid.
During evolution, several types of homologous units or domain structures (generally coded by individual exons) have been added to the trypsin-like protease, enabling the fibrinolytic proteases to gradually fulfill more specialized tasks. The addition of five kringle domains gave rise to plasminogen, whereas the addition of two kringles, one finger and one EGF (epidermal growth factor) domain gave rise t-PA. In similar fashion, the addition of one kringle and EGF domain gave rise to u-PA.
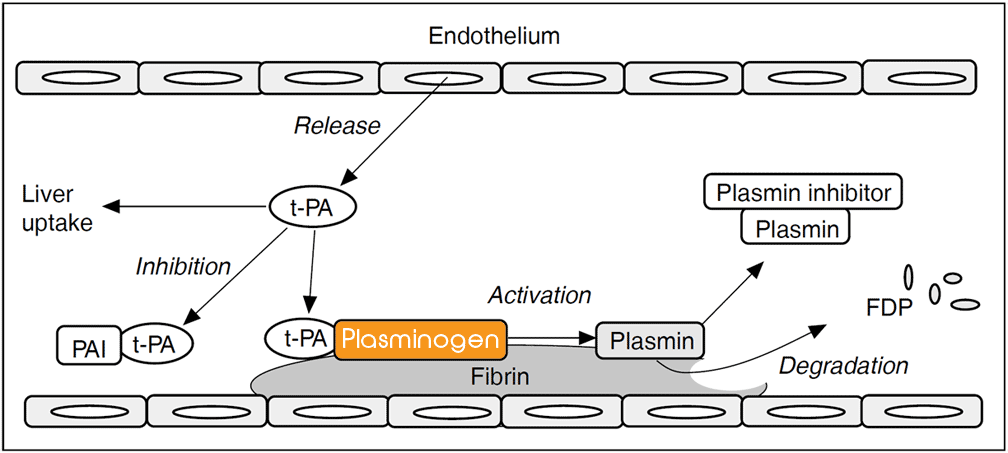
Schematic illustration of physiologic fibrinolysis. Plasminogen is the proenzyme of plasmin, whose primary target is the degradation of fibrin in the vasculature. t-PA is the principle activator of plasminogen in blood, while u-PA is the predominant activator outside the bloodstream in the extracellular matrix. t-PA is produced by the vascular endothelial cells and is released into the circulation after stimulation. Fibrin regulates its own destruction by providing receptors or binding sites for plasminogen and t-PA, thus localizing the action of plasmin. Inhibition of the system can occur at the level of plasminogen activation (PAI-1) or at the level of plasmin (plasmin inhibitor). Free t-PA as well as complexed t-PA/PAI-1 is cleared from the circulation by receptors in the liver. Abbreviations: t-PA= tissue-type plasminogen activator, PAI-1= plasminogen activator inhibitor 1, FDP= fibrin degradation products
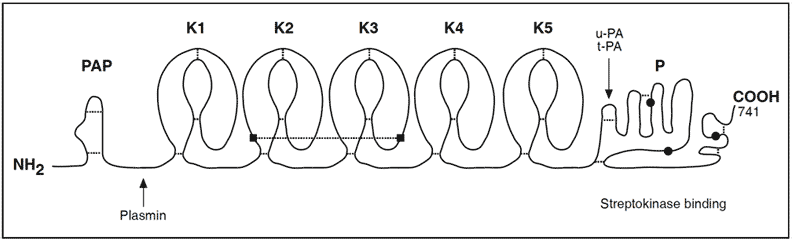
Domain structure of the human plasminogen molecule. The plasminogen molecule consists of a preactivation peptide (PAP), five kringle domains and a protease domain. The preactivation peptide is generated by plasmin cleavage giving rise to a slightly shorter plasminogen molecule called Lys-plasminogen. Activation of plasminogen into plasmin occurs when plasminogen activators (t-PA, u-PA) cleave a unique bond in the serine protease domain resulting in two polypeptide chains, linked to each other via two disulphide bonds. The three amino acid residues His603, Asp646 and Ser741 (indicated by circles) are part of the active site pocket. Kringles 2 and 3 are connected by an inter-kringle disulfide bond.
Plasminogen
Human plasminogen is a single-chain glycoprotein containing 791 amino acid residues and 2% carbohydrate. Its molecular mass is about 92,000 daltons. The plasminogen molecule contains a total of six structural domains, each with different properties. The N-terminal portion of the molecule consists of five kringle domains with the capacity to bind to fibrin. The kringle domain was first described by Magnussen et al (1975) who compared the structure with Danish pastry. Together with the preactivation peptide, the kringles control the ability of plasminogen to adopt different conformations. The protease domain resembles that of other serine proteases and contains the active site pocket His603, Asp646 and Ser741. This region also contains Ala601 which appears to be essential for the normal function of plasminogen, since mutation to Thr601 leads to an increased risk of thrombosis.
Several forms of plasminogen in plasma are known and can be separated by affinity chromatography. The native form of plasminogen in plasma has glutamic acid at the N-terminal and is termed Glu-plasminogen. Other plasminogen forms generated by the catalytic cleavage by plasmin and containing mostly lysine at the N-terminal position, are termed Lys-plasminogen.
Glu-plasminogen exists in a closed conformation that becomes extended when binding to lysine residues on a fibrin surface. A similar conformational change is believed to take place when Glu-plasminogen is converted to Lys-plasminogen. The physiological role of these conformational changes is not well known although the general effect is believed to be an increased plasminogen activation rate catalyzed by t-PA. The opposite effect is observed in the presence of anions, in particular with Cl-, which stabilizes the closed form of Glu-plasminogen rendering plasminogen poorly activatable.
Plasmin
Activation of plasminogen by its natural activators, t-PA and u-PA, involves a bond cleavage at a specific site in the plasminogen molecule, which gives rise to a two-chain molecule linked by two disulphide bonds. The plasmin formed may degrade fibrin(ogen) in a variety of ways resulting in soluble fibrin degradation products or fragments called X,Y,D and E. Plasmin is a relatively non-specific protease and can degrade not only fibrin but also many proteins in both plasma and extracellular spaces. In the coagulation pathway factors V, VIII, and von Willebrand factor are known targets of plasmin.
Plasmin activity is inhibited mainly by binding to the plasmin inhibitor, which forms a stable complex with plasmin devoid of proteolytic activity.
Plasminogen activators
Plasminogen activators, can be divided into two groups: endogenous activators (t-PA and u-PA), present in blood and other body fluids, and exogenous activators (e.g. streptokinase). Plasminogen activators are used as clot-dissolving (thrombolytic) agents for the treatment of pulmonary embolism and acute myocardial infarction.
t-PA
Tissue-type plasminogen activator (t-PA) is the principal endogenous activator of plasminogen in blood. It is produced as a single-chain molecule by the vascular endothelial cells and is secreted into the plasma continuously or by an acute release reaction following stimulation of certain endothelial cell receptors. Rapid fluctuations in t-PA concentration can be observed in response to exercise, venous occlusion, alcohol and drugs, such as DDAVP and anabolic steroids. Individuals who do not show increased t-PA activity when exposed to some of these stimuli, may be a risk group for deep vein thrombosis.
Plasmin cleavage of t-PA produces a more active two-chain molecule. However, unlike many other serine proteases, t-PA is active in its single-chain form, especially in the presence of fibrin or fibrin(ogen) fragments.
Single-chain t-PA is a 68 kDa glycoprotein, consisting of 530 amino acids and containing 7-13% carbohydrate. In human plasma, t-PA occurs mainly as a complex together with its principal inhibitor PAI-1. The level of t-PA antigen is about 5 mg/ml, whereas the concentration of free t-PA is only about 1 mg/l or 0.5 IU/ml (specific activity range 500,000 to 700,000 U/mg). The single-chain t-PA molecule is converted by plasmin to a two-chain form by cleavage of the Arg275-Ile276 peptide bond. Binding to fibrin concentrates and correctly orientates t-PA and plasminogen, as well as inducing conformational changes in the molecules that promote efficient clot lysis.
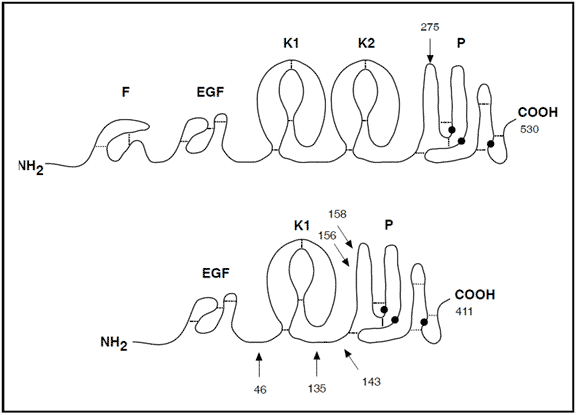
Domain structures of t-PA and u-PA. The t-PA molecule is composed of at least five domains: a finger domain, the epidermal growth factor domain (EGF), two kringle domains and the protease domain. The u-PA molecule consists of a EGF domain, one kringle and the protease domain. The finger domain is homologous to structures found in fibronectin. In the t-PA molecule this domain is implicated with fibrin binding. The EGF domain often confers affinity to specific receptors on cell surfaces.
Urokinase
Urokinase-type plasminogen activator (u-PA) is mainly produced in the kidneys as an inactive single-chain molecule (scu-PA). u-PA has its major function in tissue-related proteolysis and is believed to play only a secondary role to t-PA as a physiological activator in blood.
The activation of scu-PA by catalytic amounts of plasmin results in a two-chain structure with increased activity towards plasminogen. Through this mechanism, initial traces of plasmin may catalyze the production of active u-PA, leading to the formation of more plasmin. u-PA can only activate plasminogen in the presence of fibrin. However, it does not bind to fibrin and is not activated by fibrin. In human plasma, u-PA antigen concentrations range from 2 to 7 ng/ml. Higher values are often found in subjects with liver cirrhosis and hepatoma.
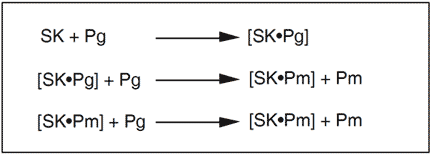
Streptokinase
Streptokinase (SK) is an exogenous plasminogen activator of 47 kDa, derived from streptococci bacteria. It is not an enzyme and functions by forming a stoichiometric 1:1 complex with human plasminogen.This complex can function as an activator of other plasminogen molecules. Complex formation is accompanied by a conformational change in the plasminogen molecule, exposing the active site to activate a second plasminogen molecule and is followed by the conversion of the SK plasminogen into a SK-plasmin complex. Both types of SK-complexes are equally efficient activators of plasminogen.
Plasminogen Aspects
Plasminogen levels
Plasminogen is synthesized in the liver and is maintained in plasma at a stable concentration of around 200 mg/l. The reference interval for plasminogen activity is 75% to 135%. In full-term neonates the plasminogen concentrations are about half those of adults, with levels gradually rising to normal by 6 months. Plasminogen levels vary little through adult life in relation to age, sex and smoking habits. There is no diurnal variation and levels are not affected by exercise. Histidine-rich glycoprotein (HRG) and plasmin inhibitor are two plasma proteins that form reversible complexes with the lysine-binding sites of plasminogen. Approximately 50% of plasminogen is bound to histidine-rich glycoprotein and about 15% to the plasmin inhibitor. Complexing with these proteins has an inhibitory effect on the binding of plasminogen to fibrin. This means that the plasma concentration of free plasminogen is determined not only by plasminogen levels but also by HRG and plasmin inhibitor. In some cases increased HRG levels may be associated with the increased risk of thrombosis.
Situations associated with decreased or elevated plasminogen levels.
Decreased plasminogen levels
- Argentine hemorrhagic fever
- DIC
- Elevated HRG
- Hereditary plasminogen deficiencies
- Hyaline membrane disease
- Hyperthyroidism
- Leukemia
- Liver disease
- Neonates
- Sepsis
- Thrombolytic therapy
Elevated plasminogen levels
- African males
- Anabolic steroids
- Hypothyroidism
- Hormonal contraceptives
- L-asparaginase therapy
- Obesity
- Post-operative surgery
- Pregnancy
- Transplant rejection
Elevated plasminogen
Elevated levels have been reported in conjunction with pregnancy (around 140% in the 2nd and 3rd trimester), hormonal contraceptives, obesity, anabolic steroids, in Africans, hypothyroidism, and in liver or kidney transplant subjects. Increased levels are usually found 3 days before any signs of transplant rejection are noticed.
Decreased plasminogen
Decreased levels have been shown in several conditions, including disseminated intravascular coagulation (DIC), sepsis, leukemia, hyaline membrane disease, liver disease, Argentine hemorrhagic fever, hyperthyroidism, and after Lasparaginase therapy, thrombolytic therapy and surgery. The decrease in plasminogen in some of these conditions may be a negative prognostic sign.
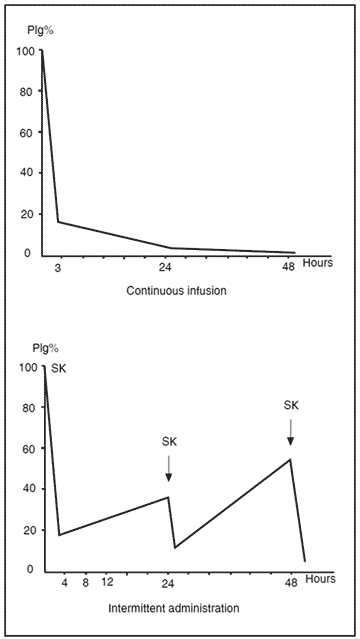
There are several mechanisms that may cause an acquired plasminogen deficiency. Increased consumption as well as depressed synthesis may be the reason for the deficiency observed in severe liver disease. An excessive release of natural plasminogen activators induced by massive stimuli (extensive tissue damage, stress, shock, certain drugs, etc.) could possibly lead to a depleted plasminogen level. The degradation of plasminogen into low molecularplasminogen by leukocyte elastase is believed to be the reason for the reduction in functional plasminogen observed in septic subjects and Argentine hemorrhagic fever.
Thrombolytic therapy
During thrombolytic therapy with high doses of streptokinase or urokinase there is a depletion of plasminogen that may terminate the efficacy of the thrombolytic drugs. The use of thrombolytic agents should therefore always involve close monitoring of the components of the plasminogen- plasmin system, especially during long-term thrombolytic treatments.
Hereditary plasminogen deficiency
There are two types of hereditary plasminogen deficiencies– hypoplasminogenemias, characterized by a parallel reduction of both plasminogen antigen and activity to about 50% of normal, and dysplasminogenemia, with a reduced activity/antigen ratio.
Hypoplasminogenemia
Hypoplasminogenemia or type I plasminogen deficiency was first reported by Hasegawa et al in 1982. Up to now, about 15 families with this disorder have been identified. Several reports indicate that subjects with hereditary plasminogen deficiency may have a higher risk of developing thrombosis. However, the correlation between type I deficiency and the risk of developing thrombosis has not yet been fully confirmed.
Dysplasminogenemia
The first case of hereditary plasminogen deficiency due to abnormalities in the plasminogen molecule and associated with venous thrombosis was reported by Aoki in 1978, and has since then been followed by similar reports.
Dysplasminogenemia or type II plasminogen deficency has been observed mainly in young males who suffer from thromboembolic disorders. In contrast to the experience with recognized thrombophilias, family members with the same abnormality do not often present with thrombosis. This fact has raised significant doubt as to whether type II plasminogen deficiency alone is a sufficient risk factor for thrombosis or whether it is only a predisposing factor in the development of thrombophilia.
Prevalence
It has been estimated that type I and II deficiency accounts for 2-3% of unexplained deep venous thrombosis in young subjects (<45 years).
The incidence of plasminogen deficiency in 1,479 healthy japanese blood donors was found to be 5.4% (plasminogen activity <60%).48 3.3% were classified as type II deficiency as they had activity antigen ratios less than 0.66. It was calculated from this data that the total number of type II deficiency in Japan was approximately 4 million out of 120 million inhabitants. In spite of this high incidence the occurrence of venous thrombosis in the Japanese population is very rare compared to Caucasians. In a recent study of 9,611 healthy Scottish blood donors, a wide range of plasminogen (25-200%) was identified. Plasminogen activity below 65% was recorded in 0.6% (61 cases), although none appeared to have a history of thrombosis. These studies suggest that plasminogen in the majority of individuals is present in excess of physiological requirements and that plasminogen deficiency is not an independent risk factor for thrombosis.
Plasminogen Assay Methods
Plasminogen assays
The concentration of plasminogen in a sample may be assayed by means of an immunoassay such as radial immunodiffusion, immunoelectrophoresis or radioimmunoassay. A common approach when screening for hereditary plasminogen deficiency is to employ a functional assay as a first-line test and, in the event of a subnormal result, follow this with an immunological assay. These two results together help distinguish between type I deficiency, when both activity and antigen levels are reduced in parallel, and type II deficiency when antigen levels are normal although activity levels are reduced. For routine purposes spectrophotometric assays based on chromogenic substrates are preferred, as they measure the functional activity of activated plasminogen and are quick to perform.
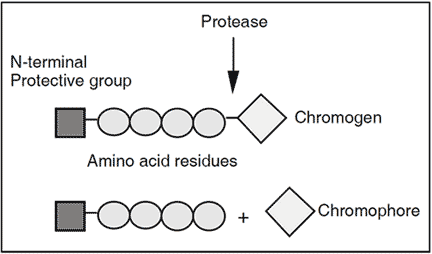
Chromogenic substrates
Chromogenic substrates for coagulation and fibrinolytic enzymes were first synthesized in the early 1970s and were soon utilized in assays for a variety of applications including plasminogen determination. A chromogenic substrate is composed of 3-5 amino acids that mimic the cleavage site of the natural protein substrate and has the chromophore 4-nitroaniline (pNA) attached to its end. When pNA is attached to the peptide chain it is colorless but when liberated by enzymatic cleavage the free pNA is yellow.
Chromogenix S-2403™ <Glu-Phe-Lys-pNA
Chromogenix S-2251™ H-D-Val-Leu-Lys-pNA
Chromogenic plasminogen assays
The determination of plasminogen using chromogenic substrates requires all plasminogen in the sample to be activated. Most assays use the streptokinase-plasminogen complex as an activator, which is formed in plasma when streptokinase is added in excess. The major advantage using this activator is that it is not inhibited by the plasmin inhibitor. A widely used chromogenic substrate for plasminogen determination is S-2251™, which is a substrate for both plasmin and the streptokinase-plasminogen complex.
When a plasmin-sensitive chromogenic substrate is incubated with the streptokinase-plasminogen complex it is cleaved and pNA is liberated. The release is measured at 405 nm, either continously during the reaction in a photometer cuvette, or after stopping the reaction with acetic or citric acid. The photometric signal is directly proportional to the plasmin activity, which reflects the amount of functional plasminogen originally present in the sample.
An important aspect to be observed when measuring the plasminogen concentration using streptokinase as an activator, is the overestimation that may occur in subjects with elevated fibrin(ogen) degradation products (FDP) which stimulate the assay reaction. The source of error can be overcome by the addition of plasminogen-free human fibrinogen in excess, thereby achieving the maximum stimulation of the streptokinase-plasminogen complex for all samples. The two major forms of plasminogen in plasma (i.e. Glu-plasminogen and Lys-plasminogen) can be measured using a chromogenic method based on the different rate of activation of the two forms of urokinase.
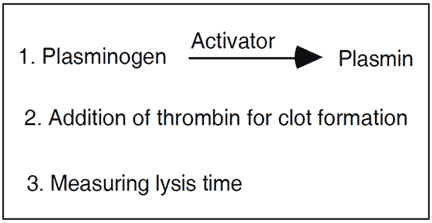
Clot lysis time
The activity of plasminogen can also be determined by means of various coagulation tests with casein or fibrin as the substrate. A typical assay would involve activation of plasminogen to plasmin by streptokinase or u-PA, the addition of plasminogen-free thrombin and measuring the lysis time. The principle is very simple although it has some major disadvantages, due mainly to the interference from the plasmin inhibitor which rapidly inactivates plasmin.
Immunological assays
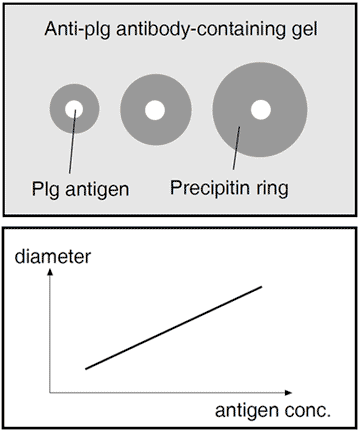
The concentration of plasminogen can be measured by its antigenicity. Since the normal antigen concentration of plasminogen is between 150 and 250 ng/l the test can be performed using simple methods with moderate sensitivity such as radial immunodiffusion. The main disadvantage is the 24-hour time lapse before results can be obtained. More rapid and sensitive methods are immunoelectrophoresis or laser nephelometry, with results obtained within 2 hours. A radioimmunoassay can also be used, which has a very high level of sensitivity but requires the handling of radioactive substances.
Radial immunodiffusion. Wells are punched in plates with agar gel containing anti-plasminogen antibody. Standard volumes of test antigen of different concentration are put in the wells. The plates are left for 24 hours, during which time the antigen diffuses out of the wells to form soluble complexes with the antibody. These continue to diffuse outwards, binding more antibody until an equivalence point is reached and the complexes precipitate in a ring. The area within the ring is proportional to the antigen concentration. Unknowns are derived by interpolation from the standard curve.